One-shot manufacturing
Saving energy and reducing CO2 emissions are high on the industrial agenda. In the mobile sectors, this means reducing the weight of components, products and systems in order to decrease the energy consumption for driving them. Weight reduction can be achieved, for instance, by replacing metal parts with parts made of lightweight materials. In this respect, textile composites are a promising class of materials, which can be used to create complex structured products with high functional value. The challenge, however, is to ensure that their mechanical properties are maintained at the level of the corresponding metal products.
The manufacturing of fiber-reinforced composite products with a thermoplastic or thermosetting polymer matrix usually involves assembling a number of separate simple parts into a single complex one. This process is labour intensive, time consuming and prone to structural failure, in particular near the interfaces between constituent parts. The EU-funded project MAPICC 3D was aimed at developing a comprehensive, one-shot manufacturing process for 3D composite preforms that avoids all these assembly steps, thus decreasing production time and increasing yield. Specifically, textile composite parts were produced using advanced weaving technology to create affordable and reliable functional products.
To demonstrate the feasibility of the manufacturing process, MAPICC 3D project members developed prototype products for various industries. For example, Coexpair created a lightweight F-shaped composite structure made of warp-interlocked woven carbon fibers, replacing a metal connection system for attaching floor panels to cabin walls in aircraft construction. Typically, bending stresses can be introduced to the connecting parts (L-brackets) and they can suffer from peeling stresses at the interface with the floor. The design of the F-shape (basically 2 L-brackets on top of each other) in the project was aimed at reducing these stresses. Reden participated in the project in order to develop modelling tools and procedures for predicting the final properties of the designed 3D composite preforms to enable easier design of customized end-products.

Simulation of stiffness and strength
Composites usually consist of continuous fibers, for example carbon or glass, arranged in a textile material and the whole embedded in a resin material such as epoxy. Unidirectional laminates can be created with all fibers aligned in one direction, or woven structures with fibers in transverse directions. In any case, the result is a highly anisotropic material that looks very different at the micro, meso and macro level.
Our challenge was to combine the micro, meso and macro level in one comprehensive composite simulation. Modelling the complete microstructure in a macroscopic model would require far too much computational resources. On the other hand, using material properties homogenized at the unit cell level would greatly reduce computational effort. This, however, meant that information about the microscale would be acquired at the macroscale and too much detail would be lost. To combine the best of both worlds we used a voxel-cell-based approach in our composite simulations. A voxel cell is an element in a mesh imposed on the macro structure and gets assigned homogenized properties.
We used our proprietary weaving simulations to predict the microstructure of the local yarn (composed of filaments) in the reinforcing woven fabric. By detecting the filaments of the yarn in the voxels, the microstructure in one voxel cell can be determined with regard to the material properties, the fiber volume fraction and the fiber directions. The homogenized material properties derived from this analysis can be subsequently used in the composite simulation at macro level, incorporating the effect of the weaving process (type of weave, process parameters and type of yarn used). Ultimately, the simulation reflects the interaction between microstructure, meso-level weaving specifics and macroscopic shape as determined by the mould that is used in production.

Validation of voxel cell approach
To validate our approach, we first conducted test simulations. For various fiber volume fraction values (representing fabric weaves that were compressed to varying degrees), we determined the composite properties. This required input from the microstructure of the yarns. We subjected a unit cell of the woven macrostructure to mechanical and thermal loads in order to determine mechanical behavior in the longitudinal and the transverse direction with respect to fiber orientation. Comparison of the results obtained with complete microstructure simulation and with our voxel-cell approach showed good overall agreement.
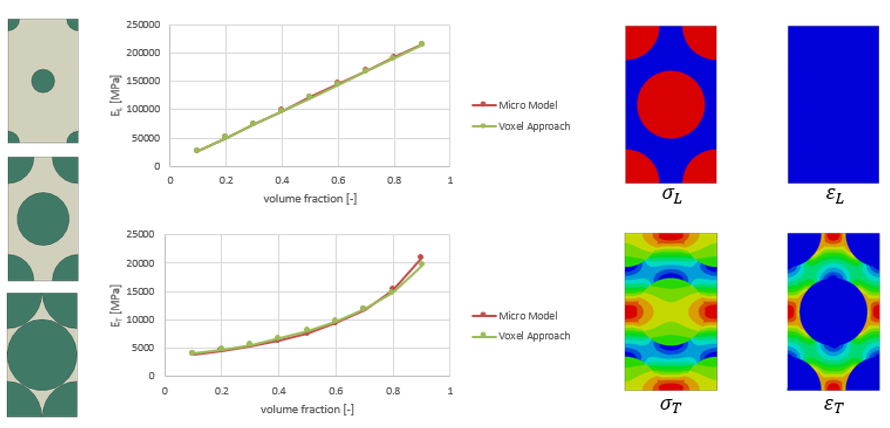
In this way, we were able to calculate stiffness, strength and effective thermal expansion of the local microstructure for varying volume fractions. For predicting the strength of the composite material, we had to include failure behavior in the equation. To this end, we selected Puck’s theory, one of the theories investigated in the World Wide Failure Exercise. This theory distinguishes between fiber failure and inter-fiber failure (i.e. of the resin material) and uses the so-called stress exposure factor to determine the stress level at which failure will occur. Strength data for calculating this factor can be obtained from either experiment or simulation. We conducted micromechanical model simulations to determine strength data for our voxel-cell approach. This provided the input for applying Puck’s theory in the post-processing of voxel-cell simulation results.
In the MAPICC 3D project, experimental tests were performed in which coupons were subjected to tension, bending and shear as well as pull-through and shear tests with bolts, while we conducted similar simulation experiments. In general, we found good agreement with experiment but also identified room for refinement. A highly relevant observation from the simulations, in line with practical experience, was that inter-fiber failure precedes fiber failure, which underlines that embedding the fibers in the resin matrix is the critical production step.
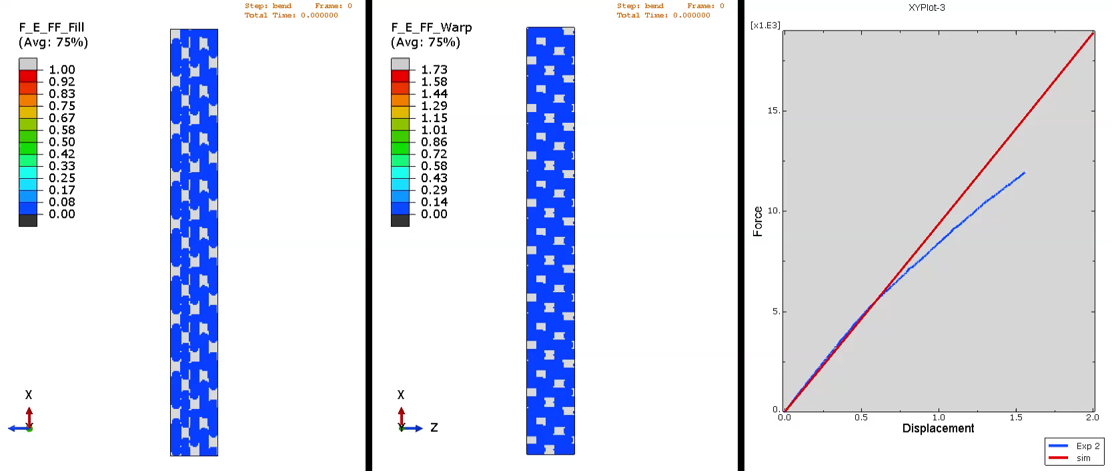
F-shape simulations
The encouraging results gave us the confidence to perform fully-fledged simulations of the aforementioned F-shaped product. This posed quite a challenge because of the sharp corners, the merging of two branches into the base of the F, and the presence of a hole for bolting. First, we created a preform using our weaving simulation. Stresses due to the consolidation of the composite were included in the simulations by incorporating curing and cooling of the product. The resulting preform was used as the input for our voxel-cell-based simulation. In a final run, we bolted two F-shapes together back-to-back, inserted metal plates and subjected the complete assembly to a pull-through test, which yielded realistic results.
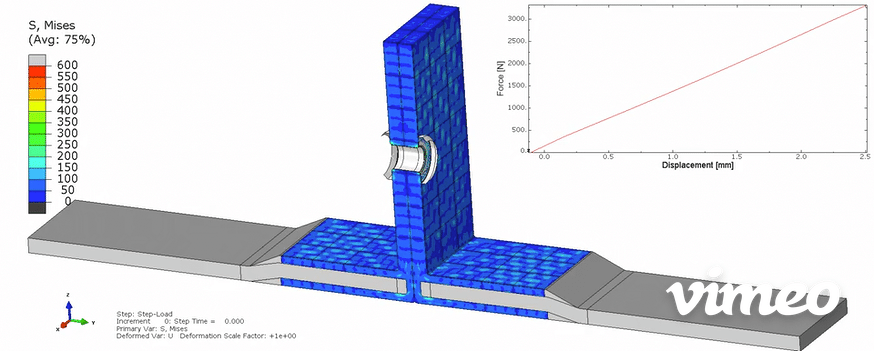
All-in all, our voxel-cell simulation approach proved to be successful in predicting stiffness as well as strength, the latter by applying Puck’s theory to assess the damage due to failure. Of course, new simulation challenges also emerged, such as incorporating stiffness degradation due to damage. But in the end, we succeeded in making the task of simulating lightweight composite structures lighter.
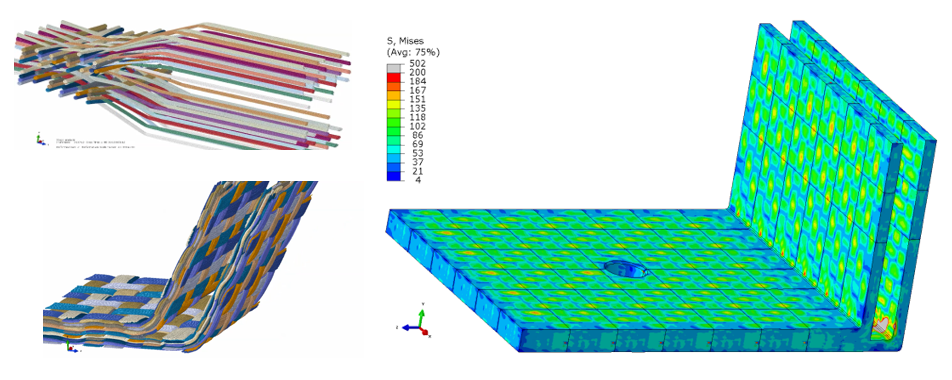
Acknowledgements
